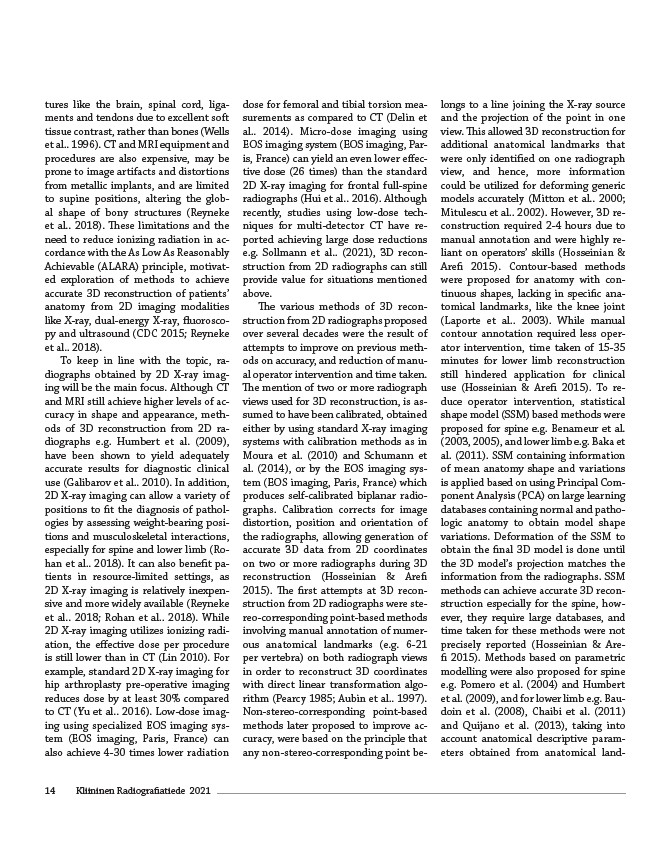
tures like the brain, spinal cord, liga-ments
and tendons due to excellent soft
tissue contrast, rather than bones (Wells
et al.. 1996). CT and MRI equipment and
procedures are also expensive, may be
prone to image artifacts and distortions
from metallic implants, and are limited
to supine positions, altering the glob-al
shape of bony structures (Reyneke
et al.. 2018). These limitations and the
need to reduce ionizing radiation in ac-cordance
with the As Low As Reasonably
Achievable (ALARA) principle, motivat-ed
exploration of methods to achieve
accurate 3D reconstruction of patients’
anatomy from 2D imaging modalities
like X-ray, dual-energy X-ray, fluorosco-py
and ultrasound (CDC 2015; Reyneke
et al.. 2018).
To keep in line with the topic, ra-diographs
obtained by 2D X-ray imag-ing
will be the main focus. Although CT
and MRI still achieve higher levels of ac-curacy
in shape and appearance, meth-ods
of 3D reconstruction from 2D ra-diographs
e.g. Humbert et al. (2009),
have been shown to yield adequately
accurate results for diagnostic clinical
use (Galibarov et al.. 2010). In addition,
2D X-ray imaging can allow a variety of
positions to fit the diagnosis of pathol-ogies
by assessing weight-bearing posi-tions
and musculoskeletal interactions,
especially for spine and lower limb (Ro-han
et al.. 2018). It can also benefit pa-tients
in resource-limited settings, as
2D X-ray imaging is relatively inexpen-sive
and more widely available (Reyneke
et al.. 2018; Rohan et al.. 2018). While
2D X-ray imaging utilizes ionizing radi-ation,
the effective dose per procedure
is still lower than in CT (Lin 2010). For
example, standard 2D X-ray imaging for
hip arthroplasty pre-operative imaging
reduces dose by at least 30% compared
to CT (Yu et al.. 2016). Low-dose imag-ing
using specialized EOS imaging sys-tem
(EOS imaging, Paris, France) can
also achieve 4-30 times lower radiation
14 Kliininen Radiografiatiede 2021
dose for femoral and tibial torsion mea-surements
as compared to CT (Delin et
al.. 2014). Micro-dose imaging using
EOS imaging system (EOS imaging, Par-is,
France) can yield an even lower effec-tive
dose (26 times) than the standard
2D X-ray imaging for frontal full-spine
radiographs (Hui et al.. 2016). Although
recently, studies using low-dose tech-niques
for multi-detector CT have re-ported
achieving large dose reductions
e.g. Sollmann et al.. (2021), 3D recon-struction
from 2D radiographs can still
provide value for situations mentioned
above.
The various methods of 3D recon-struction
from 2D radiographs proposed
over several decades were the result of
attempts to improve on previous meth-ods
on accuracy, and reduction of manu-al
operator intervention and time taken.
The mention of two or more radiograph
views used for 3D reconstruction, is as-sumed
to have been calibrated, obtained
either by using standard X-ray imaging
systems with calibration methods as in
Moura et al. (2010) and Schumann et
al. (2014), or by the EOS imaging sys-tem
(EOS imaging, Paris, France) which
produces self-calibrated biplanar radio-graphs.
Calibration corrects for image
distortion, position and orientation of
the radiographs, allowing generation of
accurate 3D data from 2D coordinates
on two or more radiographs during 3D
reconstruction (Hosseinian & Arefi
2015). The first attempts at 3D recon-struction
from 2D radiographs were ste-reo-
corresponding point-based methods
involving manual annotation of numer-ous
anatomical landmarks (e.g. 6-21
per vertebra) on both radiograph views
in order to reconstruct 3D coordinates
with direct linear transformation algo-rithm
(Pearcy 1985; Aubin et al.. 1997).
Non-stereo-corresponding point-based
methods later proposed to improve ac-curacy,
were based on the principle that
any non-stereo-corresponding point be-longs
to a line joining the X-ray source
and the projection of the point in one
view. This allowed 3D reconstruction for
additional anatomical landmarks that
were only identified on one radiograph
view, and hence, more information
could be utilized for deforming generic
models accurately (Mitton et al.. 2000;
Mitulescu et al.. 2002). However, 3D re-construction
required 2-4 hours due to
manual annotation and were highly re-liant
on operators’ skills (Hosseinian &
Arefi 2015). Contour-based methods
were proposed for anatomy with con-tinuous
shapes, lacking in specific ana-tomical
landmarks, like the knee joint
(Laporte et al.. 2003). While manual
contour annotation required less oper-ator
intervention, time taken of 15-35
minutes for lower limb reconstruction
still hindered application for clinical
use (Hosseinian & Arefi 2015). To re-duce
operator intervention, statistical
shape model (SSM) based methods were
proposed for spine e.g. Benameur et al.
(2003, 2005), and lower limb e.g. Baka et
al. (2011). SSM containing information
of mean anatomy shape and variations
is applied based on using Principal Com-ponent
Analysis (PCA) on large learning
databases containing normal and patho-logic
anatomy to obtain model shape
variations. Deformation of the SSM to
obtain the final 3D model is done until
the 3D model’s projection matches the
information from the radiographs. SSM
methods can achieve accurate 3D recon-struction
especially for the spine, how-ever,
they require large databases, and
time taken for these methods were not
precisely reported (Hosseinian & Are-fi
2015). Methods based on parametric
modelling were also proposed for spine
e.g. Pomero et al. (2004) and Humbert
et al. (2009), and for lower limb e.g. Bau-doin
et al. (2008), Chaibi et al. (2011)
and Quijano et al. (2013), taking into
account anatomical descriptive param-eters
obtained from anatomical land-